53. O2 and CO2 transport in the body
Oxygen transport in the blood
99.7% of the oxygen transported in blood is transported by binding to haemoglobin. The remaining 0,3% is dissolved in the plasma. Each molecule of Hb can bind 4 molecules of O2.
We can measure the O2 “content” of the blood in two ways. First, we can measure the percentage of all haemoglobin molecules in the blood that are completely saturated with O2. This is called the oxygen saturation.
Secondly can we measure the partial pressure of O2 (pO2) in the blood. This is measured in mmHg, or millimetres of mercury, a unit of pressure. The normal values for oxygen and carbon dioxide content in the blood is shown in this table:
Oxygen saturation | Oxygen partial pressure (pO2) | Carbon dioxide partial pressure (pCO2) | |
Arterial blood | 95 – 100% | 65 – 100 mmHg | 40 mmHg |
Venous blood | 70 – 75% | 40 mmHg | 46 mmHg |
The relationship between the oxygen partial pressure and the oxygen saturation can be shown in a haemoglobin oxygen dissociation curve.
Haemoglobin oxygen dissociation curve
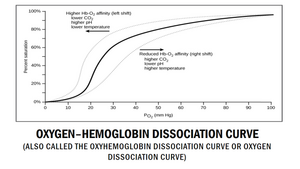
The reason behind the sigmoid shape of the curve has to do with how haemoglobin changes when it binds to oxygen. Binding of the first O2 molecule to haemoglobin changes the structure of haemoglobin slightly, so that the second O2 molecule will bind more easily. The same occurs with the third and fourth O2 molecules. This means that as haemoglobin becomes more and more saturated its affinity to O2 increases, making it easier for haemoglobin to saturate completely.
As an example on how to read the curve, we can see that when the pO2 is around 40 mmHg, around 70% of all haemoglobin molecules saturated with O2, which fits with the table above. Note that the relationship is not linear, so that a 10% decrease in pO2 doesn’t mean a 10% decrease in saturation.
Shifting of the oxygen-haemoglobin dissociation curve:
Several factors can “shift” the curve to the left or right. For reasons that you don’t have to understand will a “right shift” of the haemoglobin oxygen dissociation curve mean that oxygen binds less easily to haemoglobin. A right shift therefore means that haemoglobin more easily gives off O2 to tissues that need it, which is a good thing. A left shift means that haemoglobin less easily gives off O2 to tissues, which is not good. A right shift is sometimes called the Bohr effect.
As seen on the picture several factors can cause this right shift. Increased temperature, increased levels of a molecule called 2,3-DPG, increased [H+] and increased pCO2 will all cause right shift of the curve.
The reason the haemoglobin oxygen dissociation curve shifts at all is to regulate which tissues receive more oxygen. The main tissue to think about here is muscle. In rest muscle doesn’t need too much oxygen, so the curve can be shifted to the left. However, when the muscles are working, they need more oxygen. They also produce metabolic by-products like H+, CO2 and heat. All three of these by-products cause the curve to shift to the right, which causes more oxygen to reach the working muscle.
2,3-DPG stands for 2,3-diphosphoglycerate (sometimes called 2,3-BPG as bisphosphoglycerate). This is a molecule that RBCs synthesize in conditions where the body is hypoxic over long time, such as in people who live in high altitudes or in certain diseases like airway obstruction or heart failure.
Conversely can we have a left shift, which isn’t really a good thing and is caused by the opposite of what causes the right-shift. It occurs when a tissue doesn’t require so much oxygen, like muscles in rest.
Carbon dioxide transport in the blood
Unlike oxygen, carbon dioxide (CO2) has no own transport molecule in the blood. 70% of CO2 in the blood is transported as bicarbonate (HCO3–), 20% is transported as bound to haemoglobin and the remaining 10% is dissolved in the blood.
CO2 transport as bicarbonate:
RBCs contain an enzyme called carbonic anhydrase. This enzyme catalyses the following reaction
CO2 + H2O <–> H2CO3
In other words, it combines CO2 and H2O to form carbonic acid (H2CO3). However, carbonic acid in the blood exists in a chemical equilibrium that looks like this:
H2CO3 <–> H+ + HCO3–
So, when CO2 enters the blood it will quickly react with water and be converted into carbonic acid, thanks to carbonic anhydrase. Some carbonic acid will then non-enzymatically be converted into bicarbonate.
This bicarbonate will travel with the blood until it reaches the lungs. In the lungs the partial pressure of CO2 is much lower. This will cause bicarbonate to be converted back into carbonic acid and which will be converted back into CO2 with the help of carbonic anhydrase. CO2 will then diffuse from the blood into the alveoli, from where it is exhaled out.
CO2 transport as bound to haemoglobin:
As mentioned, 20% of carbon dioxide in the blood is transported with haemoglobin. When CO2 binds to haemoglobin it doesn’t bind to the same binding site as O2 does. In other words, both CO2 and O2 can be bound to the same haemoglobin molecule at the same time. However, when CO2 binds to haemoglobin it is converted into another form of haemoglobin called carbaminohaemoglobin. Carbaminohaemoglobin has lower affinity for O2 than normal haemoglobin.
Remember from the table above that the partial pressure of CO2 in arterial blood is 40 mmHg while it is 46 mmHg in venous blood. Note that there is only a small difference, unlike for O2. Even arterial blood contains a lot of CO2.